In the modern cardiac catheterization laboratory, technological and procedural innovations increasingly permit the performance of ever more cognitively and technically ‘complex’ and ‘high-risk’ percutaneous coronary interventions (PCI).1 Decades of stepwise advances in interventional technology, techniques, and pharmacology have improved the safety and efficacy of PCI in progressively more complicated patient, anatomic, and lesion subsets. Safe and effective circulatory and ventricular hemodynamic support may also now allow the appropriate application of complex PCI to more comorbid and older high-risk patient populations who might not otherwise be eligible for intervention.2,3
High-risk Versus Complex
High-risk and/or complex PCI may or may not coexist in an individual patient – and are not synonymous. The term, ‘complex,’ typically encompasses both lesion characteristics as well as advanced procedural skills and equipment – whereas ‘high-risk’ is usually patient-focused, and is determined primarily by comorbidities and clinical status.4 At the dawn of PCI, all percutaneous interventions were considered high risk, regardless of complexity. The initial US experience published by the National Heart, Lung, and Blood Institute Registry reported procedural PCI success rates of <60%, and emergent coronary artery bypass surgery rates >6% during the performance of interventions that would be considered non-complex by current standards.5,6
Anatomically or procedurally complex interventions may include PCI involving the distal left main (LM) coronary artery, vein grafts, at a bifurcation or trifurcation, in a heavily calcified coronary artery, in-stent interventions for re-stenosis or occlusion, or coronary chronic total occlusions – and often require specialized mental decision-making, equipment, and technical expertise. A procedure may be considered high-risk in the presence of several anatomic features and/or patient comorbidities and clinical conditions to include, but not limited to, last-remaining vessel or LM coronary artery (particularly in left-dominant systems or with a concomitant right coronary artery chronic total occlusion) supplying a large myocardial territory, severe valvular disease, severely reduced ejection fraction (EF), decompensated heart failure or cardiogenic shock, significant peripheral vascular disease, pulmonary hypertension or advanced lung disease, significant kidney or liver disease, and clinical frailty. Often, many of these complex and high-risk characteristics travel together and comprise the modern complex high-risk (CHIP) patient–lesion–procedure triad – which may warrant consideration of adjunctive mechanical circulatory support (MCS) to prevent severe adverse periprocedural hemodynamic perturbations.4
Recently, the British Cardiovascular Intervention Society database of PCI procedures performed in the UK from 2006 to 2016 was examined to better define CHIP PCI. Variables associated with in-hospital major adverse cardiac or cardiovascular events were identified and used to construct a CHIP score. Several patient factors (age ≥80 years, female sex, previous stroke, previous MI, vascular disease, EF <30%, and renal disease) and procedural factors (rotational atherectomy, LM or 3-vessel PCI, dual arterial access, use of mechanical support, and lesion length >60 mm) were associated with in-hospital major adverse cardiac and cerebrovascular events, and defined as markers of both complexity and risk.7 In accordance with other international analyses, the mean CHIP score not surprisingly increased significantly during the 2006–2016 analysis period.7

Multiple methods have been previously validated to determine patient outcomes with surgical and percutaneous coronary revascularization to include clinical systems, such as the Society of Thoracic Surgeons, European System for Cardiac Operative Risk Evaluation, and National Cardiovascular Data Registry (NCDR) CathPCI registry risk scores.8–10 Integrated anatomic and clinical scores, such as the SYNTAX score and the SYNTAX II 2020 update, provide additional value in assessing comparative mortality rates of PCI and coronary artery bypass (CABG).11,12 Unfortunately, historical risk scores have proven less concordant at higher levels of procedural risk – although newer scoring systems may overcome some of these limitations.7,13–15 CHIP patients declined for surgical revascularization based on elevated surgical risk and/or severe medical comorbidities represent a particularly high-risk subgroup – with mortality rates out of proportion to that assessed by traditional PCI risk stratification tools.16,17
Rationale for Mechanical Circulatory Support Use
In clinical practice, decisions regarding the choice of medical therapy alone, PCI, or CABG require a tailored multidisciplinary team-based and patient-centered approach that integrates clinical factors, procedural considerations, operator and institution issues, clinical trial data, and clinical practice guidelines (Figure 1). Overall, the purpose of a comprehensive pre-procedural assessment is to identify clinical, anatomic, and procedural factors most favorable or unfavorable for short- and long-term outcomes for medical therapy, PCI, and CABG, and best align physician and patient objectives. Similarly integrated risk–benefit decision-making is required to determine which patients may or may not benefit most from MCS use for non-emergent high-risk PCI (HRPCI), and which device may be most safe and effective.
Left ventricular systolic dysfunction, decompensated heart failure, and other adverse hemodynamic factors increase the short-term risks of both surgical and percutaneous approaches – and are typically key determinants of consideration of elective MCS use. Although PCI is generally well-tolerated in most patients, repeated procedural myocardial ischemia may result in significant reductions in cardiac output and increases in cardiac filling pressures, which may be poorly tolerated in patients with little or no physiologic reserve.18 Maximally developed left ventricular (LV) pressure may fall below mean arterial pressure – after which the heart no longer ejects blood, the aortic valve no longer opens, and systemic circulation is no longer maintained.19 MCS devices may prevent this cascade of hemodynamic instability, and facilitate more complete and effective revascularization.
Recent data from 302 patients undergoing MCS-supported HRPCI enrolled in the non-randomized PROTECT III observational study treated at 38 US sites between 2017 and 2020 demonstrated that hemodynamic status at the time of HRPCI, as opposed to patient comorbidities or anatomic complexity, was most associated with loss of pulsatility, transient dependence on MCS devices for circulatory support, and increased rates of major adverse cardiac and cerebrovascular events – demonstrating the significance of ventricular dysfunction and the value of intraprocedural invasive hemodynamic monitoring.20
Observational-only studies demonstrate improved procedural cardiovascular hemodynamics and sometimes more complete revascularization with MCS use, despite higher-risk patient profiles.21 Additional non-randomized data demonstrate survival benefit and reduced rates of major bleeding when an up-front prophylactic MCS strategy is employed compared with intra- or post-procedure bailout use.22,23 Most recently, a non-randomized Premier Healthcare Database propensity-adjusted observational analysis demonstrated that Impella use during non-emergent HRPCI may be associated with improved survival and reduced in-hospital MI compared with intra-aortic balloon pump (IABP).24
MCS use may also serve as an ‘auto-pilot,’ and provide background ventricular and circulatory stability to permit an interventional cardiologist ‘pilot’ to perform safe multitasking while simultaneously performing and/or supervising sedation and anesthesia, intracoronary imaging, hemodynamic assessment and management, and PCI. The risk–benefit analysis of MCS use may also evolve with operator and center annual and lifetime volume and experience (and thus vary from one institution to another). Finally, considerations for or against elective MCS use for HRPCI may vary by country and region – often dependent on available resources.25
Current State of Affairs
The evidence for use of MCS in high-risk non-emergent PCI is limited and presently inconclusive (Table 1). One recent analysis examined 2,108,715 consecutive patients with stable coronary artery disease (CAD) undergoing elective PCI in the CathPCI registry between 2009 and 2018, and noted an increase in MCS use from 0.2% in 2009 to 0.6% in 2018.26 IABP was the predominant device in this analysis and was associated with higher MACE (9.6% versus 6.0%), but lower risk of complications (18.2% versus 19.1%) compared with non-IABP MCS. The main high-risk features associated with prophylactic MCS use were unprotected LM PCI, use of atherectomy, and EF <30%. The MCS group had higher rates of previous heart failure, diabetes, lung disease, three-vessel disease, and the presence of three or more high-risk features together.
In a non-randomized observational analysis of 1,598 CTO PCIs performed from 2012 to 2017 at 12 high-volume US centers, a MCS device was used electively in 4% of procedures and urgently in 1% of procedures. Patients with elective MCS use had higher prevalence of heart failure, lesion calcification, J-CTO scores, retrograde intervention, and lower EF (34 ± 14% versus 50 ± 14%; p<0.001). Despite more complex clinical and angiographic characteristics, elective use of MCS in these high-risk patients demonstrated similar technical and procedural success rates, but with higher risk of complications, compared with cases without elective MCS.27
The 2021 American College of Cardiology/American Heart Association/Society for Cardiovascular Angiography and Interventions Guidelines for Coronary Artery Revascularization presently provides a class 1 level of evidence B recommendation for surgical revascularization to improve survival in patients with stable ischemic heart disease and multivessel CAD with severe LV systolic dysfunction, and notes that there may be an advantage of PCI over medical therapy in patients who have a clinical indication for CABG, but are deemed a prohibitive surgical risk.28 The document further provides a class 2B level of evidence B recommendation for elective insertion of a hemodynamic support device as an adjunct to prevent hemodynamic compromise during PCI in these patients.
One contemporary analysis of 1,013 patients undergoing non-emergent PCI for unprotected LM or multivessel CAD demonstrated that 22% were deemed ineligible for surgical revascularization.16 Several observational reports have demonstrated favorable outcomes with PCI for surgically ineligible patients compared with those predicted for CABG.29,30 In one single-center report, 342 of 1,363 patients successfully underwent coronary revascularization (80% PCI, 13% CABG, 7% hybrid surgical–percutaneous) after a median interval of 2.2 years from a diagnosis of ‘unrevascularizable’ CAD.30 More recently, preliminary results of the prospective OPTIMUM Registry examining outcomes of 750 patients across 22 US centers deemed ineligible for surgery presented at Transcatheter Cardiovascular Therapeutics 2021 demonstrated favorable early outcomes (5.6% observed rate of death at 30 days) with PCI compared with surgical estimates (10.4% predicted risk of death) with CABG.31
A contemporary US NCDR assessment of unprotected LM (ULM) PCI demonstrated that the mean annual ULM PCI volume was 0.5 procedures per operator, and only 16% of operators performed an average of one or more ULM PCI annually.32 Another NCDR analysis revealed that the median annual number of total PCI procedures performed per operator in the US was 59, and 44% of operators performed <50 total PCI procedures per year.33 In a separate study, an experienced, high-volume ULM PCI operator was defined as one who performed at least 15 ULM PCIs per year for at least three consecutive years – and noted that this was correlated with reduced 30-day and 3-year mortality.34 A similar analysis of the NCDR CathPCI Registry identified distinct operator profiles (based on case volume, anatomic complexity, and use of atherectomy, intracoronary diagnostics, and mechanical circulatory support) that are differentially associated with patient outcomes.35 Yet another study demonstrated that operator volume is an important factor in determining outcome after ULM PCI – and a lower threshold of minimum operator ULM PCI volume associated with improved survival was ≥16 cases/year.36 Across multiple observational analyses, an increase in both operator and institutional volume of all-comer and CHIP PCI has been associated with a decrease in adverse outcomes, length of hospital stay, and cost of hospitalization – and should probably be factored into decision-making for CHIP interventions.37,38
Contemporary Mechanical Circulatory Support Devices
The ideal MCS device permits easy percutaneous implantation, effective and reliable circulatory support and ventricular unloading, is simple to manage post-insertion, has very low complication rates, and offers simple and safe mechanisms for device removal, vascular closure, and hemostasis. Unfortunately, no ‘perfect’ device presently exists, and the sum of current evidence in favor of MCS includes limited randomized data, small sample sizes, primarily surrogate outcomes, no comparisons with medical or surgical therapy, and typically short-term follow-up.
Studies evaluating the use of adjunctive MCS devices during HRPCI have reported mixed results. The BCIS-1 trial demonstrated that IABP use led to fewer procedural complications (compared with no support) during HRPCI with no difference in the primary endpoint of major adverse cardiovascular events at 28 days.39 In the PROTECT II trial, >65% of patients had class III/IV heart failure, the average EF was 24%, and 63% of patients were deemed surgically inoperable.40 The composite primary endpoint of major adverse events at 30 days was similar between the IABP and Impella arms (40.1% versus 35.1%; p=0.28). However, on per-protocol analysis, there appeared to be a benefit with Impella for the composite endpoint at 90 days (51.0% versus 40.0%; p=0.023), driven mostly by a reduction in the need for repeat revascularization (8.1% versus 3.7%; p=0.06).
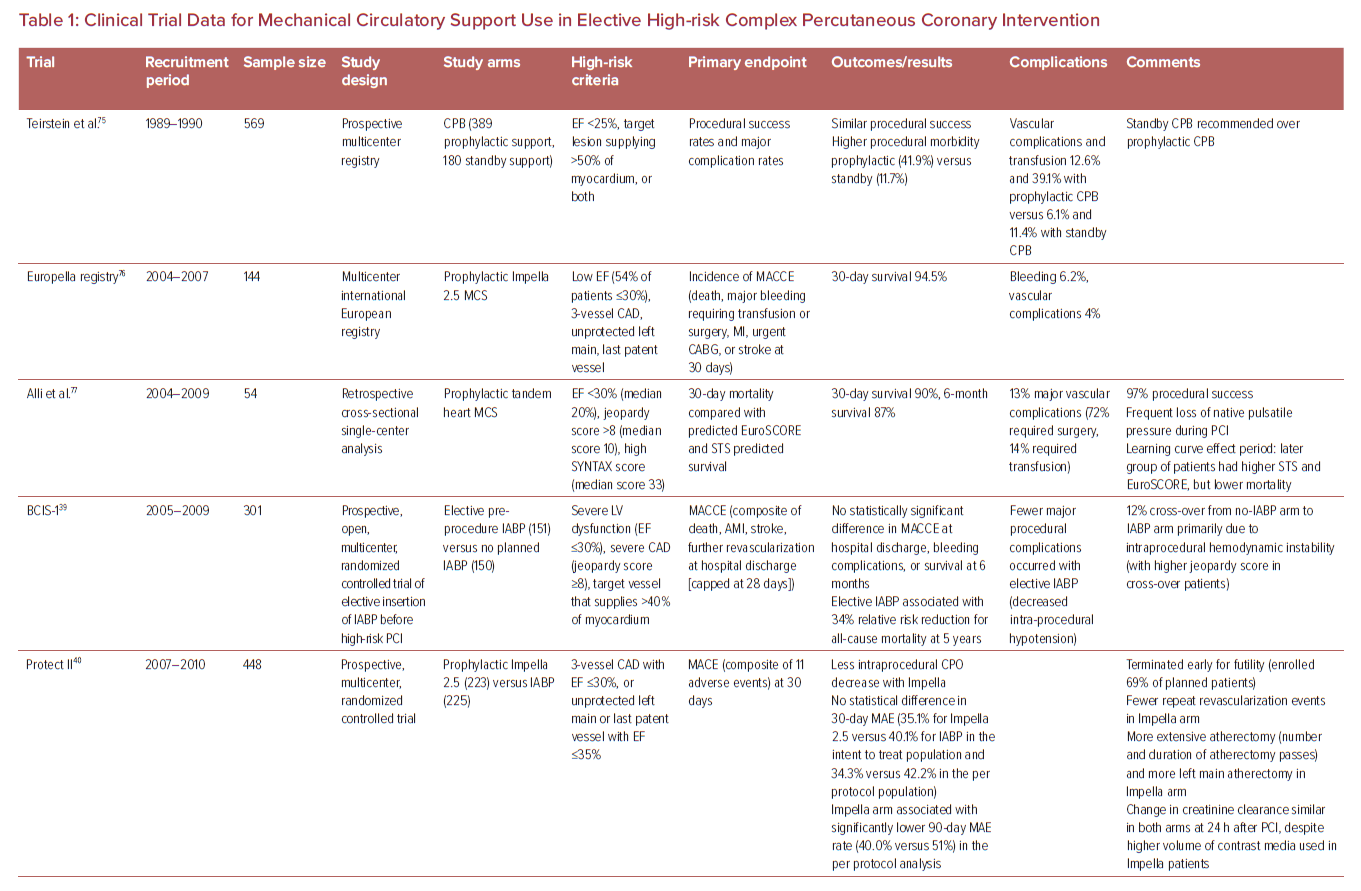

MCS devices can be divided into those that support the LV, right ventricle, or both, and those that provide oxygenation support or not. The most commonly used devices to support CHIP procedures are the LV support devices. Percutaneous MCS devices currently in clinical use in the US are summarized in Figure 2 and include:
IABP:
- Requires a non-large-bore 7–8 Fr sheath – providing a safe option for patients with small peripheral vasculature and/or significant peripheral artery disease.
- Only provides an estimated 0.5–1 l/min of support.
- As a counterpulsation device, relies in part on intact underlying native heart pulsation – thereby limiting its effectiveness in the setting of severe ventricular dysfunction.41
Impella:
- Impella CP requires a 14 Fr large-bore sheath most commonly inserted via the femoral or axillary artery.
- The Impella CP device can provide support up to 3.5 l/min and also effectively lowers LV end-diastolic pressure, which may improve coronary perfusion in the presence of critical coronary artery stenoses.42
- The 5.0 and 5.5 devices (providing 5.0 and 5.5 l/min output) are typically placed surgically via an axillary conduit – and require a 21 Fr sheath (although transcaval implantation has been described).43
TandemHeart:
- Uses an extracorporeal centrifugal pump to create a left atrium to femoral artery bypass circuit and commonly provides support >4 l/min.
- Arterial cannula sizes range from 15 to 19 Fr – with high rates of distal limb ischemia as Fr size increases.
- Requires experience in transseptal puncture and either transesophageal or intracardiac echocardiography – which may not be readily available at all times in all centers.
- Suited to use in the presence of LV thrombus, severe aortic stenosis, and/or a mechanical aortic valve.
Venoarterial extracorporeal membrane oxygenation (ECMO):
- Provides maximal biventricular support and oxygenation support.
- Support advantages come at a higher rate of bleeding and vascular complications.44
- Standard ultrasound-guided percutaneous arterial and venous cannulation.
- Complex follow-on intensive care unit indwelling management – requiring trained and certified perfusionists.45
Risks and Complications
Balancing benefits and risks when offering invasive therapies is an integral part of caring for patients with complex CAD. The significantly elevated risks of vascular and bleeding complications (Figure 3) with all current MCS devices should be factored into decision-making and assessment of relative risks of PCI with or without MCS compared with CABG or medical therapy only.46–48 A recent analysis of non-IABP percutaneous ventricular assist device use for HRPCI from 2008 to 2018 demonstrated a 27-fold increase in use across a patient population with a higher burden of comorbidities and with increased complexity of interventions (higher proportion of atherectomy and multivessel intervention) – with lower vascular complication rates over time (from 6% to 4%) and without a significant difference in mortality.49 If MCS devices are used, appropriate training and best practices for ultrasound-guided large-bore access are paramount, and optimal efficient PCI practices to minimize ischemic time during interventions are crucial.1,50,51
IABP has been associated with bleeding, vascular, and non-vascular complications.52,53 An improperly positioned or too large IABP can result in decreased gastrointestinal, renal, or cerebral perfusion.54 Thrombus or emboli may result in cerebrovascular accident or abdominal visceral ischemia.55 Non-vascular complications include thrombocytopenia (from deposition of platelets on the balloon and mechanical destruction of thrombocytes) and hemolysis (from disruption of erythrocytes). Finally, balloon rupture, while rare, may also occur.56,57
When compared with IABP, there is a significant increase in bleeding and vascular complications with the larger-bore Impella devices. There is also an elevated risk of hemolysis that may contribute to both acute kidney injury and the need for blood transfusion – although these events more typically occur with longer-term use in shock (versus CHIP procedures). Device migration may also result in injury to the aortic valve or mitral valve (or subvalvular apparatus) necessitating surgical repair or replacement.58
TandemHeart complications are mostly similar to other MCS devices. However, unique complications related to the trans-septal puncture include air embolism, cardiac tamponade, and atrial perforation.59 Significant right-to-left shunting may also result if there is dislodgement of the inflow cannula from the left to the right atrium.60 In some cases, a residual atrial septal defect may later necessitate closure.61
Venoarterial extracorporeal ECMO carries the highest complication rates of the MCS devices. Bleeding with ECMO is common, is not limited to cannulation sites, and may involve the brain and gastrointestinal tract. Risks of lower extremity ischemia may be reduced with the use of an obligate distal perfusion cannula.62 Other major risks of ECMO include LV distention secondary to retrograde blood flow and loss of cardiac pulsatility – with resultant pulmonary edema and/or intracardiac thrombus.63 North–south syndrome, resulting in hypoxia of the head and upper extremities, may also occur.64
Decision Algorithms
All high-risk and/or complex PCI should occur on a background of optimized disease-modifying guideline-directed medical therapy, such as lifestyle modification, antiplatelet and antihyperlipidemic therapy, blood pressure and diabetes control, and cardiomyopathy medication optimization (e.g. β-blocker, mineralocorticoid receptor antagonist, angiotensin receptor/neprilysin inhibitor, and sodium–glucose cotransporter 2 inhibitor).65 Decision algorithms to use a MCS device (or not) during PCI typically incorporate the anatomic complexity, area of myocardium to be treated or at risk, underlying chronic and acute cardiac and systemic hemodynamic states, presence of significant valvular disease and other major medical comorbidities, surgical eligibility, operator and center expertise, and peripheral vascular anatomy.46

Age, comorbidity, and clinical frailty often travel together, affect both surgical and percutaneous outcomes, and should be considered in conjunction with traditional risk factors when counseling patients regarding the periprocedural risks associated with percutaneous or surgical therapies.66 Integrating a multidisciplinary heart team into institutional practice provides a formalized approach to evaluating complex and high-risk CAD – and may reduce within and between facility differences, and best ensures standardized decision-making and management, and equitable care delivery.67 This may include the development and implementation of evaluation and management algorithms and protocols, quality metrics, data collection and tracking for ongoing quality improvement, patient education, and outreach and communication with referring physicians and facilities. To best facilitate these objectives and lead this initiative, a dedicated non-physician CHIP coordinator should be strongly considered.68
Management decisions in the increasing population of older patients are particularly challenging. Older (and frail) adults are more often focused on nearer-term risks and outcomes, such as recovery time, stroke or neurocognitive decline, and physical debility, and may thus favor less-invasive therapeutic options.69,70 Despite the established benefits of coronary revascularization with or without MCS, medical therapy alone may be preferred if the weight of comorbidities is high, life expectancy is short, or the likelihood of benefit is low. In some instances, palliative care may be most appropriate.
The importance of appropriate patient selection is further highlighted by a recent analysis of 1,674 patients in the Veterans Affairs Health Care System who died within 30 days of their PCI procedure between 2005 and 2016 – demonstrating that a minority of deaths (28%) were due to cardiovascular causes and only a very small proportion (8%) were attributable directly to the procedure.71
Future Directions
With rapid advancements in the field of interventional cardiology, we are able to treat a wide spectrum of coronary artery disease percutaneously. There remains a large population of patients who may be declined for revascularization due to comorbidities, surgical ineligibility, complexity of coronary anatomy, or adverse hemodynamics. A risk–treatment paradox may exist where the highest-risk patients with the greatest potential benefit may simultaneously be the least likely to be offered treatment. As procedural risk increases, more advanced skillsets are required to achieve high technical and procedural success rates. There exists a need for standardized advanced coronary training, maintenance of procedural volume and complexity, and lifelong learning as new skills and technologies emerge.4,51,72 It remains to be determined whether high-risk and complex PCI safety and success could be improved by selective referral to experienced operators at experienced centers.
Multiple real-world studies and comparative effectiveness analyses have been limited by measured and unmeasured confounding. Long-term clinical follow-up data regarding survival, ventricular recovery, heart failure hospitalizations, or target lesion revascularization are unfortunately missing from most studies. Looking ahead, prospective randomized trials are the standard by which the safety and efficacy of MCS for use in HRPCI will be judged. Hopefully in-progress randomized trials, such as PROTECT IV (NCT04763200) and CHIP-BCIS3 (NCT05003817), that compare outcomes between MCS-assisted and MCS-unassisted PCI in patients with complex CAD and impaired LV function will offer more definitive safety and efficacy data, and better identify key comorbidity, hemodynamic, anatomic, and procedural factors to better guide clinical decision-making for the use (and type) of prophylactic MCS devices for elective HRPCI in stable CAD. The PROTECT IV trial will also include a right heart catheterization substudy – which should additionally provide important hemodynamic decision-making data.
Conclusion
Adverse events in CHIP are high compared with non-high-risk interventions – which serves as an important reminder that the non-surgical option is not risk-free. Appropriate use of MCS devices is an important part of this equation. Absent randomized data with similar patient demographics, comorbidities, anatomy, and hemodynamics undergoing similar PCI procedures – but with different or no MCS devices – make it difficult to disentangle adverse cardiovascular events related to the patient, the procedure, or the operator/center (or to compare outcomes with medical therapy only or with surgery).71
While waiting for further evidence, we may therefore be well-served by integrating a formal multidisciplinary heart team model into institutional practice to best ensure standardized and equitable patient evaluation and care delivery, optimize patient hemodynamics pre-PCI with best contemporary medical therapy (which may eliminate any need for MCS in many instances), and carefully assess and weigh the relative risks of both MCS-assisted and MCS-unassisted PCI compared with medical therapy, surgical revascularization, or durable left ventricular assist device implantation or heart transplantation.1,73,74 Ongoing research to best identify the patients that may derive the greatest benefit from MCS-assisted HRPCI is also needed. Technological innovations to include lower-profile devices that maximize hemodynamic benefits while minimizing vascular complications will be additionally critical to optimizing safety–efficacy trade-offs.
While most contemporary PCI by far does not necessitate any consideration of hemodynamic support, and more high-quality and randomized evidence is surely needed, there is likely middle ground between the ‘therapeutic nihilism’ and ‘irrational exuberance’ of both extreme sides of the argument. In the end, for the time being, the complex decision-making process of whether or not to use prophylactic MCS may never be wholly algorithmic, but should be approached in a thoughtful and considered multidisciplinary, team-based, patient-centered, and evidence-based manner.